Group A Streptococcal (GAS) Infections Treatment & Management. Антибиотики streptococcus pyogenes
Streptococcus pyogenes - Wikipedia
Streptococcus pyogenes is a species of Gram-positive bacteria. These bacteria are aerotolerant and an extracellular bacterium, made up of non-motile and non-sporing cocci. As expected with a streptococci, it is clinically important in human illness. It is an infrequent, but usually pathogenic, part of the skin microbiota. It is the predominant species harboring the Lancefield group A antigen, and is often called group A streptococcus (GAS). However, both Streptococcus dysgalactiae and the Streptococcus anginosus group can possess group A antigen. Group A streptococci when grown on blood agar typically produces small zones of beta-hemolysis, a complete destruction of red blood cells. (A zone size of 2–3 mm is typical.) It is thus also called group A (beta-hemolytic) streptococcus (GABHS), and can make colonies greater than 5 mm in size.[1] Like other cocci, streptococci are round bacteria. The name is derived from Greek words meaning chain(Strepto) of berries (coccus) and pus(pyo)-forming(genes), because streptococcal cells tend to link in chains of round cells (see image) and a number of infections caused by the bacterium, produce pus. Streptococci are catalase-negative.[2]S. pyogenes can be cultured on blood agar plates. Under ideal conditions, it has an incubation period of 1 to 3 days.[3]
An estimated 700 million GAS infections occur worldwide each year. While the overall mortality rate for these infections is 0.1%, over 650,000 of the cases are severe and invasive, and have a mortality rate of 25%.[4] Early recognition and treatment are critical; diagnostic failure can result in sepsis and death.[5][6] Epidemiology[edit] The S. pyogenes typically colonises the throat, genital mucosa, rectum, and skin. 1% to 5% of healthy individuals have throat, vaginal or rectal carriage. While in healthy children, such carriage rate varies from 2% to 17%. There are four methods for the transmission of this bacteria: inhalation of respiratory droplets, skin contact, contact with objects, suface, or dust that is contaminated with bacteria or less commonly transmission through food. Such bacteria can cause a variety of diseases such as streptococcal pharyngitis, rheumatic fever, rheumatic heart disease, and scarlet fever. Although pharyngitis are mostly bacterial in origin, about 15 to 30% of all pharyngitis cases in children are streptococcal; meanwhile, 5 to 20% of pharyngitis in adults are streptococcal. The number of pharyngitis cases is higher in children when compared to adults due to exposures in schools, nurseries and lower host immunity. Such cases Streptococcal pharyngitis occurs more frequently from December to April (later winter to early spring) in seasonal countries, possibly due to changing climate, behavioural changes or predisposing viral infection. Disease cases is the lowest during autumn.[7]
MT1 (metabolic type 1) clone is frequently associated with invasive Streptococcus pyogenes infections among developed countries. The incidence and mortality of S. pyognes was high during the pre-penicillin era, but started to fall prior to penicillin widespread availability. Therefore, environmental factor do play a role in the S. pyogenes infection. Incidence of S. pyogenes is 2 to 4 per 100,000 population in developed countries and 12 to 83 per 100,000 population in developed countries. S. pyogenes infection is more frequently found in men when compared to women, with highest rates in elderly and then followed by infants. In people with risk factors such as heart disease, diabetes, malignancy, blunt trauma, surgical incision, viral respiratory infection, and influenza, S. pyogenes infection happens in 17 to 25% of all cases. It usually happens within one week of the diagnosis of influenza infection. In children S. pyogenes infection, 14 to 16% of the cases have prior chickenpox infection. Such S. pyogenes infection in children is usually manifested as severe soft tissue infection with duration of onset of 4 to 12 days from the day of chickenpox diagnosis. There is also 40 to 60 times increase in risk of S. pyogenes infection within the first two weeks of chickenpox infection in children. However, 20 to 30% of S. pyogenes infection do occur in adults with no identifiable risk factors. The incidence is higher in children (50 to 80% of S. pyogenes infection) with no known risk factors. The rates of scarlet fever in UK was usually 4 in 100,000 population, however, in 2014, the rates had risen to 49 per 100,000 population. Rheumatic fever and rheumatic heart disease (RHD) usually occurs at 2 to 3 weeks after the throat infection, which is more common among the impoverish people in developing countries. From 1967 to 1996, the global mean incidence of rheumatic fever and RHD was 19 per 100,000 with the highest incidence at 51 per 100,000.[7]
Maternal S. pyogenes infection usually happens in the late pregnancy at more than 30 weeks of gestation to 4 weeks post pregnancy, which accounts for 2 to 4% of all the S. pyogenes infections. This represents 20 to 100 times increase in risk for S. pyogenes infections. Clinical manifestations are: pneumonia, septic arthritis, necrotizing fasciitis, and genital tract sepsis. According to a study done by Queen Charlotte’s hospital in London during the 1930s, vagina was not the common source of such infection. On the contrary, maternal throat and the close contacts were the more common sites for maternal S. pyogenes infection.[7] Bacteriology[edit] Serotyping[edit] In 1928, Rebecca Lancefield published a method for serotyping S. pyogenes based on its M protein,[8] a virulence factor displayed on its surface.[9] Later, in 1946, Lancefield described the serologic classification of S. pyogenes isolates based on their surface T-antigen.[10] Four of the 20 T-antigens have been revealed to be pili, which are used by bacteria to attach to host cells.[11] As of 2016, a total of 120 M proteins are identified. These M proteins are encoded by 234 types emm gene with greater than 1,200 alleles.[7]
Virulence factors[edit] S. pyogenes has several virulence factors that enable it to attach to host tissues, evade the immune response, and spread by penetrating host tissue layers.[12] A carbohydrate-based bacterial capsule composed of hyaluronic acid surrounds the bacterium, protecting it from phagocytosis by neutrophils.[2] In addition, the capsule and several factors embedded in the cell wall, including M protein, lipoteichoic acid, and protein F (SfbI) facilitate attachment to various host cells.[13] M protein also inhibits opsonization by the alternative complement pathway by binding to host complement regulators. The M protein found on some serotypes is also able to prevent opsonization by binding to fibrinogen.[2] However, the M protein is also the weakest point in this pathogen's defense, as antibodies produced by the immune system against M protein target the bacteria for engulfment by phagocytes. M proteins are unique to each strain, and identification can be used clinically to confirm the strain causing an infection.[14]
Lysogeny[edit] All strains of S. pyogenes are polylysogenized, in that they carry one or more bacteriophage on their genomes.[15] Some of the phages may be defective, but in some cases active phage may compensate for defects in others.[16] In general, the genome of S. pyogenes strains isolated during disease are >90% identical, they differ by the phage they carry.[17] Name Description Streptolysin O | An exotoxin, one of the bases of the organism's beta-hemolytic property, streptolysin O causes an immune response and detection of antibodies to it; antistreptolysin O (ASO) can be clinically used to confirm a recent infection. | Streptolysin S | A cardiotoxic exotoxin, another beta-hemolytic component, not immunogenic and O2 stable: A potent cell poison affecting many types of cell including neutrophils, platelets, and subcellular organelles. |
Streptococcal pyrogenic exotoxin A (SpeA) | Superantigens secreted by many strains of S. pyogenes: This pyrogenic exotoxin is responsible for the rash of scarlet fever and many of the symptoms of streptococcal toxic shock syndrome, also known as toxic shock like syndrome(TSLS). | Streptococcal pyrogenic exotoxin C (SpeC) | Streptokinase | Enzymatically activates plasminogen, a proteolytic enzyme, into plasmin, which in turn digests fibrin and other proteins | Hyaluronidase | Hyaluronidase is widely assumed to facilitate the spread of the bacteria through tissues by breaking down hyaluronic acid, an important component of connective tissue. However, very few isolates of S. pyogenes are capable of secreting active hyaluronidase due to mutations in the gene that encode the enzyme. Moreover, the few isolates capable of secreting hyaluronidase do not appear to need it to spread through tissues or to cause skin lesions.[18] Thus, the true role of hyaluronidase in pathogenesis, if any, remains unknown. |
Streptodornase | Most strains of S. pyogenes secrete up to four different DNases, which are sometimes called streptodornase. The DNases protect the bacteria from being trapped in neutrophil extracellular traps (NETs) by digesting the NETs' web of DNA, to which are bound neutrophil serine proteases that can kill the bacteria.[19] | C5a peptidase | C5a peptidase cleaves a potent neutrophil chemotaxin called C5a, which is produced by the complement system.[20] C5a peptidase is necessary to minimize the influx of neutrophils early in infection as the bacteria are attempting to colonize the host's tissue.[21] C5a peptidase, although required to degrade the neutrophil chemotaxin C5a in the early stages of infection, is not required for S. pyogenes to prevent the influx of neutrophils as the bacteria spread through the fascia.[22] | Streptococcal chemokine protease | The affected tissue of patients with severe cases of necrotizing fasciitis are devoid of neutrophils.[23] The serine protease ScpC, which is released by S. pyogenes, is responsible for preventing the migration of neutrophils to the spreading infection. ScpC degrades the chemokine IL-8, which would otherwise attract neutrophils to the site of infection.[21][22] |
Genome[edit] The genome of different strains were sequenced (genome size is 1.8–1.9 Mbp)[24] encoding about 1700-1900 proteins (1700 in strain NZ131,[25][26] 1865 in strain MGAS5005[27][28]). Disease[edit] S. pyogenes is the cause of many important human diseases, ranging from mild superficial skin infections to life-threatening systemic diseases.[2] Infections typically begin in the throat or skin. The most striking sign is a strawberry-like rash. Examples of mild S. pyogenes infections include pharyngitis (strep throat) and localized skin infection (impetigo). Erysipelas and cellulitis are characterized by multiplication and lateral spread of S. pyogenes in deep layers of the skin. S. pyogenes invasion and multiplication in the fascia can lead to necrotizing fasciitis, a life-threatening condition requiring surgery.[citation needed][29] The bacterium is found in neonatal infections.[30]
Infections due to certain strains of S. pyogenes can be associated with the release of bacterial toxins. Throat infections associated with release of certain toxins lead to scarlet fever. Other toxigenic S. pyogenes infections may lead to streptococcal toxic shock syndrome, which can be life-threatening.[2] S. pyogenes can also cause disease in the form of postinfectious "nonpyogenic" (not associated with local bacterial multiplication and pus formation) syndromes. These autoimmune-mediated complications follow a small percentage of infections and include rheumatic fever and acute postinfectious glomerulonephritis. Both conditions appear several weeks following the initial streptococcal infection. Rheumatic fever is characterised by inflammation of the joints and/or heart following an episode of streptococcal pharyngitis. Acute glomerulonephritis, inflammation of the renal glomerulus, can follow streptococcal pharyngitis or skin infection. This bacterium remains acutely sensitive to penicillin. Failure of treatment with penicillin is generally attributed to other local commensal organisms producing β-lactamase, or failure to achieve adequate tissue levels in the pharynx. Certain strains have developed resistance to macrolides, tetracyclines, and clindamycin.
Applications[edit] Bionanotechnology[edit] Many S. pyogenes proteins have unique properties, which have been harnessed in recent years to produce a highly specific "superglue"[31][32] and a route to enhance the effectiveness of antibody therapy.[33] Genome editing[edit] The CRISPR system from this organism that is used to recognize and destroy DNA from invading viruses, stopping the infection, was appropriated in 2012 for use as a genome-editing tool that could potentially alter any piece of DNA and later RNA.[34] See also[edit] References[edit] - ^ "Streptococcus pyogenes - Pathogen Safety Data Sheets". Government of Canada, Public Health Agency of Canada.
- ^ a b c d e Ryan KJ, Ray CG, eds. (2004). Sherris Medical Microbiology (4th ed.). McGraw Hill. ISBN 0-8385-8529-9.
- ^ Streptococcal Pharyngitis, archived from the original on May 13, 2012
- ^ Aziz RK, Kansal R, Aronow BJ, Taylor WL, Rowe SL, Kubal M, Chhatwal GS, Walker MJ, Kotb M (2010). Ahmed N, ed. "Microevolution of Group A Streptococci In Vivo: Capturing Regulatory Networks Engaged in Sociomicrobiology, Niche Adaptation, and Hypervirulence". PLoS ONE. 5 (4): e9798. doi:10.1371/journal.pone.0009798. PMC 2854683 . PMID 20418946. Retrieved 2011-08-12.
- ^ Jim Dwyer (July 11, 2012). "An Infection, Unnoticed, Turns Unstoppable". The New York Times. Retrieved July 12, 2012.
- ^ Jim Dwyer (July 18, 2012). "After Boy's Death, Hospital Alters Discharging Procedures". The New York Times. Retrieved July 19, 2012.
- ^ a b c d Androulla, Efstratiou; Theresa, Lamagni (10 February 2016). "Epidemiology of Streptococcus pyogenes". Streptococcus pyogenes : Basic Biology to Clinical Manifestations. Oklahoma City, United States: University of Oklahoma Health Sciences Center. Retrieved 24 February 2018.
- ^ Pignanelli S, Brusa S, Pulcrano G, Catania MR, Cocchi E, Lanari M (2015). "A rare case of infant sepsis due to the emm-89 genotype of Group A Streptococcus within a community-acquired cluster". New Microbiol. 38 (4): 589–92. PMID 26485019.
- ^ Lancefield RC (1928). "The antigenic complex of Streptococcus hemolyticus". J Exp Med. 47 (1): 9–10. doi:10.1084/jem.47.1.91.
- ^ Lancefield RC, Dole VP (1946). "The properties of T antigen extracted from group A hemolytic streptococci". J Exp Med. 84 (5): 449–71. doi:10.1084/jem.84.5.449. PMC 2135665 . PMID 19871581.
- ^ Mora M, Bensi G, Capo S, Falugi F, Zingaretti C, Manetti AG, Maggi T, Taddei AR, Grandi G, Telford JL (2005). "Group A Streptococcus produce pilus-like structures containing protective antigens and Lancefield T antigens". Proc Natl Acad Sci USA. 102 (43): 15641–6. doi:10.1073/pnas.0507808102. PMC 1253647 . PMID 16223875.
- ^ Patterson MJ (1996). Baron S; et al., eds. Streptococcus. In: Baron's Medical Microbiology (4th ed.). Univ of Texas Medical Branch. ISBN 0-9631172-1-1.
- ^ Bisno AL, Brito MO, Collins CM (2003). "Molecular basis of group A streptococcal virulence". Lancet Infect Dis. 3 (4): 191–200. doi:10.1016/S1473-3099(03)00576-0. PMID 12679262.
- ^ Engel ME, Muhamed B, Whitelaw AC, Musvosvi M, Mayosi BM, Dale JB. Group A streptococcal emm type prevalence among symptomatic children in Cape Town and potential vaccine coverage. Pediatr Infect Dis J. 2014 Feb;33(2):208-10. doi: 10.1097/INF.0b013e3182a5c32a.PMID 23934204
- ^ Ferretti JJ; McShan WM; Ajdic D; Savic DJ; Savic G; Lyon K; et al. (2001). "Complete Genome Sequence of an M1 Strain of Streptococcus pyogenes". Proc Natl Acad Sci USA. 98: 4658–63. doi:10.1073/pnas.071559398. PMC 31890 . PMID 11296296.
- ^ Canchaya C, Desiere F, McShan WM, Ferretti JJ, Parkhill J, Brussow H (2002). "Genome analysis of an inducible prophage and prophage remnants integrated in the Streptococcus pyogenes strain SF370". Virology. 302: 245–58. doi:10.1006/viro.2002.1570. PMID 12441069.
- ^ Banks DJ, Porcella SF, Barbian KD, Martin JM, Musser JM (2003). "Structure and distribution of an unusual chimeric genetic element encoding macrolide resistance in phylogenetically diverse clones of group A Streptococcus". J Infect Dis. 188: 1898–908. doi:10.1086/379897. PMID 14673771.
- ^ Starr CR, Engleberg NC (2006). "Role of Hyaluronidase in Subcutaneous Spread and Growth of Group A Streptococcus". Infect Immun. 74 (1): 40–8. doi:10.1128/IAI.74.1.40-48.2006. PMC 1346594 . PMID 16368955.
- ^ Buchanan JT, Simpson AJ, Aziz RK, Liu GY, Kristian SA, Kotb M, Feramisco J, Nizet V (2006). "DNase expression allows the pathogen group A Streptococcus to escape killing in neutrophil extracellular traps". Curr Biol. 16 (4): 396–400. doi:10.1016/j.cub.2005.12.039. PMID 16488874.
- ^ Wexler DE, Chenoweth DE, Cleary PP (1985). "Mechanism of action of the group A streptococcal C5a inactivator". Proc Natl Acad Sci USA. 82 (23): 8144–8. doi:10.1073/pnas.82.23.8144. PMC 391459 . PMID 3906656.
- ^ a b Ji Y, McLandsborough L, Kondagunta A, Cleary PP (1996). "C5a peptidase alters clearance and trafficking of group A streptococci by infected mice". Infect Immun. 64 (2): 503–10. PMC 173793 . PMID 8550199.
- ^ a b Hidalgo-Grass C, Mishalian I, Dan-Goor M, Belotserkovsky I, Eran Y, Nizet V, Peled A, Hanski E (2006). "A streptococcal protease that degrades CXC chemokines and impairs bacterial clearance from infected tissues". EMBO J. 25 (19): 4628–37. doi:10.1038/sj.emboj.7601327. PMC 1589981 . PMID 16977314.
- ^ Hidalgo-Grass C, Dan-Goor M, Maly A, Eran Y, Kwinn LA, Nizet V, Ravins M, Jaffe J, Peyser A, Moses AE, Hanski E (2004). "Effect of a bacterial pheromone peptide on host chemokine degradation in group A streptococcal necrotising soft-tissue infections". Lancet. 363 (9410): 696–703. doi:10.1016/S0140-6736(04)15643-2. PMID 15001327.
- ^ Beres SB, Richter EW, Nagiec MJ, Sumby P, Porcella SF, DeLeo FR, Musser JM (2006). "Molecular genetic anatomy of inter- and intraserotype variation in the human bacterial pathogen group a Streptococcus". Proceedings of the National Academy of Sciences. 103 (18): 7059–64. doi:10.1073/pnas.0510279103. PMC 1459018 . PMID 16636287.
- ^ "Streptococcus pyogenes NZ131".
- ^ McShan, W. M.; Ferretti, J. J.; Karasawa, T; Suvorov, A. N.; Lin, S; Qin, B; Jia, H; Kenton, S; Najar, F; Wu, H; Scott, J; Roe, B. A.; Savic, D. J. (2008). "Genome sequence of a nephritogenic and highly transformable M49 strain of Streptococcus pyogenes". Journal of Bacteriology. 190 (23): 7773–85. doi:10.1128/JB.00672-08. PMC 2583620 . PMID 18820018.
- ^ Sumby, P; Porcella, S. F.; Madrigal, A. G.; Barbian, K. D.; Virtaneva, K; Ricklefs, S. M.; Sturdevant, D. E.; Graham, M. R.; Vuopio-Varkila, J; Hoe, N. P.; Musser, J. M. (2005). "Evolutionary origin and emergence of a highly successful clone of serotype M1 group a Streptococcus involved multiple horizontal gene transfer events". The Journal of Infectious Diseases. 192 (5): 771–82. doi:10.1086/432514. PMID 16088826.
- ^ "Streptococcus pyogenes MGAS5005".
- ^ "Necrotizing Fasciitis". CDC. Content source: National Center for Immunization and Respiratory Diseases, Division of Bacterial Diseases. Page maintained by: Office of the Associate Director for Communication, Digital Media Branch, Division of Public Affairs. October 26, 2017. Retrieved 2018-01-06.
- ^ Baucells, B.J.; Mercadal Hally, M.; Álvarez Sánchez, A.T.; Figueras Aloy, J. (2015). "Asociaciones de probióticos para la prevención de la enterocolitis necrosante y la reducción de la sepsis tardía y la mortalidad neonatal en recién nacidos pretérmino de menos de 1.500g: una revisión sistemática". Anales de Pediatría. doi:10.1016/j.anpedi.2015.07.038. ISSN 1695-4033.
- ^ "Flesh-eating bacteria inspire superglue - University of Oxford".
- ^ Zakeri B, Fierer JO, Celik E, Chittock EC, Schwarz-Linek U, Moy VT, Howarth M (2012). "Peptide tag forming a rapid covalent bond to a protein, through engineering a bacterial adhesin". Proceedings of the National Academy of Sciences. 109 (12): E690–7. doi:10.1073/pnas.1115485109. PMC 3311370 . PMID 22366317.
- ^ Baruah K, Bowden TA, Krishna BA, Dwek RA, Crispin M, Scanlan CN (2012). "Selective Deactivation of Serum IgG: A General Strategy for the Enhancement of Monoclonal Antibody Receptor Interactions". Journal of Molecular Biology. 420 (1–2): 1–7. doi:10.1016/j.jmb.2012.04.002. PMC 3437440 . PMID 22484364.
- ^ Zimmer, Carl (2016-06-03). "Scientists Find Form of Crispr Gene Editing With New Capabilities". The New York Times. ISSN 0362-4331. Retrieved 2016-06-10.
Further reading[edit] External links[edit] en.wikipedia.org Streptococcus - Wikipedia
Streptococcus is a genus of coccus (spherical) Gram-positive bacteria belonging to the phylum Firmicutes[4] and the order Lactobacillales (lactic acid bacteria). Cell division in this genus occurs along a single axis in these bacteria, thus they grow in chains or pairs, hence the name—from Greek στρεπτός streptos, meaning easily bent or twisted, like a chain (twisted chain). (Contrast this with staphylococci, which divide along multiple axes and generate grape-like clusters of cells.) Most are oxidase-negative and catalase-negative, and many are facultative anaerobes. In 1984, many bacteria formerly considered Streptococcus were separated out into the genera Enterococcus and Lactococcus.[5] Currently, over 50 species are recognised in this genus. This genus has been found to be part of the salivary microbiome.[6] Pathogenesis and classification[edit] In addition to streptococcal pharyngitis (strep throat), certain Streptococcus species are responsible for many cases of pink eye,[7]meningitis, bacterial pneumonia, endocarditis, erysipelas, and necrotizing fasciitis (the 'flesh-eating' bacterial infections). However, many streptococcal species are not pathogenic, and form part of the commensal human microbiota of the mouth, skin, intestine, and upper respiratory tract. Furthermore, streptococci are a necessary ingredient in producing Emmentaler ("Swiss") cheese. Species of Streptococcus are classified based on their hemolytic properties.[8] Alpha-hemolytic species cause oxidization of iron in hemoglobin molecules within red blood cells, giving it a greenish color on blood agar. Beta-hemolytic species cause complete rupture of red blood cells. On blood agar, this appears as wide areas clear of blood cells surrounding bacterial colonies. Gamma-hemolytic species cause no hemolysis. Beta-hemolytic streptococci are further classified by Lancefield grouping, a serotype classification (that is, describing specific carbohydrates present on the bacterial cell wall).[9] The 20 described serotypes are named Lancefield groups A to V (excluding I and J). In the medical setting, the most important groups are the alpha-hemolytic streptococci S. pneumoniae and Streptococcus viridans group, and the beta-hemolytic streptococci of Lancefield groups A and B (also known as “group A strep” and “group B strep”). Table: Medically relevant streptococci (not all are alpha hemolytic)[8] Species | Host | Disease | S. pyogenes | human | pharyngitis, cellulitis | S. agalactiae | human, cattle | neonatal meningitis and sepsis | S. dysgalactiae | human, animals | endocarditis, bacteremia, pneumonia, meningitis, respiratory infections | S. bovis | human, animals | biliary or urinary tract infections, endocarditis | S. anginosus | human, animals | subcutaneous/organ abscesses, meningitis, respiratory infections | S. sanguinis | human | endocarditis, dental caries | S. suis | swine | meningitis | S. mitis | human | endocarditis | S. mutans | human | dental caries | S. pneumoniae | human | pneumonia |
Alpha-hemolytic[edit] When alpha hemolysis (α-hemolysis) is present, the agar under the colony is dark and greenish. Streptococcus pneumoniae and a group of oral streptococci (Streptococcus viridans or viridans streptococci) display alpha hemolysis. This is sometimes called green hemolysis because of the color change in the agar. Other synonymous terms are incomplete hemolysis and partial hemolysis. Alpha hemolysis is caused by hydrogen peroxide produced by the bacterium, oxidizing hemoglobin to green biliverdin. Pneumococci[edit] - S. pneumoniae (sometimes called pneumococcus), is a leading cause of bacterial pneumonia and occasional etiology of otitis media, sinusitis, meningitis, and peritonitis. Inflammation is thought to be the major cause of how pneumococci cause disease, hence the tendency of diagnoses associated with them to involve inflammation.
The viridans group: alpha-hemolytic[edit] Beta-hemolytic[edit] Beta hemolysis (β-hemolysis), sometimes called complete hemolysis, is a complete lysis of red cells in the media around and under the colonies: the area appears lightened (yellow) and transparent. Streptolysin, an exotoxin, is the enzyme produced by the bacteria which causes the complete lysis of red blood cells. There are two types of streptolysin: Streptolysin O (SLO) and streptolysin S (SLS). Streptolysin O is an oxygen-sensitive cytotoxin, secreted by most Group A Streptococcus (GAS), and interacts with cholesterol in the membrane of eukaryotic cells (mainly red and white blood cells, macrophages, and platelets), and usually results in β-hemolysis under the surface of blood agar. Streptolysin S is an oxygen-stable cytotoxin also produced by most GAS strains which results in clearing on the surface of blood agar. SLS affects immune cells, including polymorphonuclear leukocytes and lymphocytes, and is thought to prevent the host immune system from clearing infection. Streptococcus pyogenes, or GAS, displays beta hemolysis. Some weakly beta-hemolytic species cause intense beta hemolysis when grown together with a strain of Staphylococcus. This is called the CAMP test. Streptococcus agalactiae displays this property. Clostridium perfringens can be identified presumptively with this test. Listeria monocytogenes is also positive on sheep's blood agar. Group A[edit] Group A S. pyogenes (GAS) is the causative agent in a wide range of group A streptococcal infections. These infections may be noninvasive or invasive. The noninvasive infections tend to be more common and less severe. The most common of these infections include streptococcal pharyngitis (strep throat) and impetigo.[10]Scarlet fever is also a noninvasive infection, but has not been as common in recent years. The invasive infections caused by group A β-hemolytic streptococci tend to be more severe and less common. This occurs when the bacterium is able to infect areas where it is not usually found, such as the blood and the organs.[11] The diseases that may be caused include streptococcal toxic shock syndrome, necrotizing fasciitis, pneumonia, and bacteremia.[10] Globally, GAS has been estimated to cause more than 500,000 deaths every year, making it one of the world's leading pathogens.[10] Additional complications may be caused by GAS, namely acute rheumatic fever and acute glomerulonephritis. Rheumatic fever, a disease that affects the joints, kidneys, and heart valves, is a consequence of untreated strep A infection caused not by the bacterium itself. Rheumatic fever is caused by the antibodies created by the immune system to fight off the infection cross-reacting with other proteins in the body. This "cross-reaction" causes the body to essentially attack itself and leads to the damage above. A similar autoimmune mechanism initiated by Group A beta-hemolytic streptococcal (GABHS) infection is hypothesized to cause pediatric autoimmune neuropsychiatric disorders associated with streptococcal infections (PANDAS), wherein autoimmune antibodies affect the basal ganglia, causing rapid onset of psychiatric, motor, sleep, and other symptoms in pediatric patients. GAS infection is generally diagnosed with a rapid strep test or by culture. Group B[edit] S. agalactiae, or group B Streptococcus, GBS, causes pneumonia and meningitis in neonates and the elderly, with occasional systemic bacteremia. They can also colonize the intestines and the female reproductive tract, increasing the risk for premature rupture of membranes during pregnancy, and transmission of the organism to the infant. The American Congress of Obstetricians and Gynecologists (formerly the American College of Obstetricians and Gynecologists), American Academy of Pediatrics, and the Centers for Disease Control recommend all pregnant women between 35 and 37 weeks gestation to be tested for GBS. Women who test positive should be given prophylactic antibiotics during labor, which will usually prevent transmission to the infant.[12] The United Kingdom has chosen to adopt a risk factor-based protocol, rather than the culture-based protocol followed in the US. Current guidelines state that if one or more of the following risk factors is present, then the woman should be treated with intrapartum antibiotics: - Preterm labour (<37 weeks)
- Prolonged rupture of membranes (>18 hours)
- Intrapartum fever (>38C)[vague]
- Prior GBS affected infante[vague]
- GBS bacteriuria during this pregnancy
This protocol results in treatment[vague] of 15–20% of pregnant women and prevention of 65–70% of cases of early onset GBS sepsis.[13] Group C[edit] This group includes S. equi, which causes strangles in horses,[14] and S. zooepidemicus—S. equi is a clonal descendent or biovar of the ancestral S. zooepidemicus—which causes infections in several species of mammals, including cattle and horses. S. dysgalactiae is also a member of group C, beta-haemolytic streptococci that can cause pharyngitis and other pyogenic infections similar to group A streptococci. Group D (enterococci)[edit] Many former group D streptococci have been reclassified and placed in the genus Enterococcus (including E. faecalis, E. faecium, E. durans, and E. avium).[15] For example, Streptococcus faecalis is now Enterococcus faecalis. E. faecalis is sometimes alpha hemolytic and E. faecium is sometimes beta hemolytic.[16] The remaining nonenterococcal group D strains include Streptococcus bovis and Streptococcus equinus. Nonhemolytic streptococci rarely cause illness. However, weakly hemolytic group D beta-hemolytic streptococci and Listeria monocytogenes (which is actually a Gram-positive bacillus) should not be confused with nonhemolytic streptococci. Group F streptococci[edit] Group F streptococci were first described in 1934 by Long and Bliss amongst the "minute haemolytic streptococci".[17] They are also known as Streptococcus anginosus (according to the Lancefield classification system) or as members of the S. milleri group (according to the European system). Group G streptococci[edit] These streptococci are usually, but not exclusively, beta-hemolytic. Streptococcus dysgalactiae is the predominant species encountered, particularly in human disease. S. canis is an example of a GGS which is typically found on animals, but can cause infection in humans. S. phocae is a GGS subspecies that has been found in marine mammals and marine fish species. In marine mammals it has been mainly associated with meningoencephalitis, septicemia, and endocarditis, but is also associated with many other pathologies. Its environmental reservoir and means of transmission in marine mammals is not well characterized. Group H streptococci[edit] Group H streptococci cause infections in medium-sized canines. Group H streptococci rarely cause illness unless a human has direct contact with the mouth of a canine. One of the most common ways this can be spread is human-to-canine, mouth-to-mouth contact. However, the canine may lick the human's hand and infection can be spread, as well.[18] Molecular taxonomy and phylogenetics[edit] Phylogenetic tree of Streptococcus species, based on data from PATRIC.[19] 16S groups are indicated by brackets and their key members are highlighted in red. Streptococci have been divided into six groups on the basis of their 16S rDNA sequences: S. anginosus, S.bovis, S. mitis, S. mutans, S. pyogenes and S. salivarius.[20] The 16S groups have been confirmed by whole genome sequencing (see figure). The important pathogens S. pneumoniae and S. pyogenes belong to the S. mitis and S. pyogenes groups, respectively[citation needed], while the causative agent of dental caries, Streptococcus mutans, is basal to the Streptococcus group. Genomics[edit] The genomes of hundreds of species have been sequenced.[22] Most Streptococcus genomes are 1.8 to 2.3 Mb in size and encode 1,700 to 2,300 proteins. Some important genomes are listed in the table.[23] The four species shown in the table (S. pyogenes, S. agalactiae, S. pneumoniae, and S. mutans) have an average pairwise protein sequence identity of about 70%.[23] feature S. pyogenes S. agalactiae S. pneumoniae S. mutans base pairs | 1,852,442 | 2,211,488 | 2,160,837 | 2,030,921 | ORFs | 1792 | 2118 | 2236 | 1963 | prophages | yes | no | no | no |
Bacteriophage[edit] Bacteriophages have been described for many species of Streptococcus. 18 prophages have been described in S. pneumoniae that range in size from 38 to 41 kb in size, encoding from 42 to 66 genes each.[citation needed] Some of the first Streptococcus phages discovered were Dp-1[24] and ω1.[25][26] In 1981 the Cp (Complutense phage) family was discovered with Cp-1 as its first member.[27] Dp-1 and Cp-1 infect both S. pneumoniae and S. mitis.[28] However, the host ranges of most Streptococcus phages have not been investigated systematically. Natural genetic transformation[edit] Natural genetic transformation involves the transfer of DNA from one bacterium to another through the surrounding medium. Transformation is a complex process dependent on expression of numerous genes. To be capable of transformation a bacterium must enter a special physiologic state referred to as competence. S. pneumoniae, S. mitis and S. oralis can become competent, and as a result actively acquire homologous DNA for transformation by a predatory fratricidal mechanism [29] This fratricidal mechanism mainly exploits non-competent siblings present in the same niche [30] Among highly competent isolates of S. pneumoniae, Li et al.[31] showed that nasal colonization fitness and virulence (lung infectivity) depend on an intact competence system. Competence may allow the streptococcal pathogen to use external homologous DNA for recombinational repair of DNA damages caused by the hosts oxidative attack [32] See also[edit] References[edit] - ^ "Result of detail taxonomy information". TXSearch Taxonomy Retrieval. DNA Data Bank of Japan. 19 February 2010. Retrieved 30 March 2010.
- ^ a b c d e f g h i j k l m n o p q r s t u v w x y z aa ab ac ad ae af ag ah ai aj ak al am an ao ap aq ar as at au av aw ax ay az ba bb bc bd be bf bg bh bi bj bk bl bm bn bo bp bq br bs bt Parte, A.C. "Streptococcus". www.bacterio.net.
- ^ LPSN entry for Streptococcus
- ^ a b Ryan KJ, Ray CG, eds. (2004). Sherris Medical Microbiology (4th ed.). McGraw Hill. pp. 293–4. ISBN 0-8385-8529-9.
- ^ Facklam R (October 2002). "What happened to the streptococci: overview of taxonomic and nomenclature changes". Clin. Microbiol. Rev. 15 (4): 613–30. doi:10.1128/CMR.15.4.613-630.2002. PMC 126867 . PMID 12364372.
- ^ Wang, Kun; Lu, Wenxin; Tu, Qichao; Ge, Yichen; He, Jinzhi; Zhou, Yu; Gou, Yaping; Nostrand, Joy D Van; Qin, Yujia; Li, Jiyao; Zhou, Jizhong; Li, Yan; Xiao, Liying; Zhou, Xuedong (10 March 2016). "Preliminary analysis of salivary microbiome and their potential roles in oral lichen planus". Scientific Reports. 6 (1). doi:10.1038/srep22943. PMC 4785528 . PMID 26961389. Retrieved 6 May 2017.
- ^ http://www.medicinenet.com/pink_eye/article.htm
- ^ a b Patterson MJ (1996). Baron S; et al., eds. Streptococcus. In: Baron's Medical Microbiology (4th ed.). Univ of Texas Medical Branch. (via NCBI Bookshelf) ISBN 0-9631172-1-1.
- ^ Facklam R (2002). "What happened to the streptococci: overview of taxonomic and nomenclature changes". Clin Microbiol Rev. 15 (4): 613–30. doi:10.1128/CMR.15.4.613-630.2002. PMC 126867 . PMID 12364372.
- ^ a b c Cohen-Poradosu R, Kasper DL (2007). "Group A streptococcus epidemiology and vaccine implications". Clin. Infect. Dis. 45 (7): 863–5. doi:10.1086/521263. PMID 17806050.
- ^ "Streptococcal Infections (Invasive Group A Strep)". New York City Department of Health and Mental Hygiene. Retrieved 21 November 2012.
- ^ Schrag S, Gorwitz R, Fultz-Butts K, Schuchat A (2002). "Prevention of perinatal group B streptococcal disease. Revised guidelines from CDC". MMWR Recomm Rep. 51 (RR-11): 1–22. PMID 12211284.
- ^ Norwitz, E.R.; Schorge, J.O. (2013). Obstetrics and Gynaecology at a Glance (4th ed.). John Wiley & Sons LTD.
- ^ Harrington DJ, Sutcliffe IC, Chanter N (2002). "The molecular basis of Streptococcus equi infection and disease". Microbes Infect. 4 (4): 501–10. doi:10.1016/S1286-4579(02)01565-4. PMID 11932201.
- ^ Köhler W (June 2007). "The present state of species within the genera Streptococcus and Enterococcus". International Journal of Medical Microbiology. 297 (3): 133–50. doi:10.1016/j.ijmm.2006.11.008. PMID 17400023.
- ^ Holt et al. (1994). Bergey's Manual of Determinative Bacteriology (9th ed.). Lippincott Williams & Wilkins. ISBN 0-683-00603-7
- ^ Whitworth JM (November 1990). "Lancefield group F and related streptococci" (PDF). J. Med. Microbiol. 33 (3): 135–51. doi:10.1099/00222615-33-3-135. PMID 2250284.
- ^ "Bacterial Infection (Streptococcus) in Dogs". petmd.com. Retrieved 12 December 2014.
- ^ "Bacteria-Firmicutes-Bacilli-Lactobacillales-Streptococcaceae-Streptococcus". PATRIC, University of Chicago. Retrieved 12 December 2014.
- ^ Kawamura Y, Hou XG, Sultana F, Miura H, Ezaki T (1995). "Determination of 16S rRNA sequences of Streptococcus mitis and Streptococcus gordonii and phylogenetic relationships among members of the genus Streptococcus". Int J Syst Bacteriol. 45: 406–408. doi:10.1099/00207713-45-2-406. PMID 7537076.
- ^ Xu, P; Alves, J. M.; Kitten, T; Brown, A; Chen, Z; Ozaki, L. S.; Manque, P; Ge, X; Serrano, M. G.; Puiu, D; Hendricks, S; Wang, Y; Chaplin, M. D.; Akan, D; Paik, S; Peterson, D. L.; MacRina, F. L.; Buck, G. A. (2007). "Genome of the opportunistic pathogen Streptococcus sanguinis". Journal of Bacteriology. 189 (8): 3166–75. doi:10.1128/JB.01808-06. PMC 1855836 . PMID 17277061.
- ^ Streptococcus genomes and related information at PATRIC
- ^ a b Ferretti JJ, Ajdic D, McShan WM (2004). "Comparative genomics of streptococcal species". The Indian journal of medical research. 119 Suppl: 1–6. PMID 15232152.
- ^ McDonnell M, Ronda C, Tomasz A (1975) “Diplophage”: a bacteriophage of Diplococcus pneumoniae. Virology 63:577–582
- ^ Tiraby JG, Tiraby E, Fox MS (1975) Pneumococcal bacteriophages. Virology 68:566–569
- ^ López R (2004). "Streptococcus pneumoniae and its bacteriophages: one long argument". Int. Microbiol. 7 (3): 163–71. PMID 15492930.
- ^ Ronda C, López R, García E (1981). "Isolation and characterization of a new bacteriophage, Cp-1, infecting Streptococcus pneumoniae". J. Virol. 40 (2): 551–9. PMC 256658 . PMID 6275103.
- ^ Ouennane S, Leprohon P, Moineau S (2015). "Diverse virulent pneumophages infect Streptococcus mitis". PLoS ONE. 10 (2): e0118807. doi:10.1371/journal.pone.0118807. PMC 4334900 . PMID 25692983.
- ^ Johnsborg O, Eldholm V, Bjørnstad ML, Håvarstein LS (2008). "A predatory mechanism dramatically increases the efficiency of lateral gene transfer in Streptococcus pneumoniae and related commensal species". Mol. Microbiol. 69 (1): 245–53. doi:10.1111/j.1365-2958.2008.06288.x. PMID 18485065.
- ^ Claverys JP, Håvarstein LS (2007). "Cannibalism and fratricide: mechanisms and raisons d'être". Nat. Rev. Microbiol. 5 (3): 219–29. doi:10.1038/nrmicro1613. PMID 17277796.
- ^ Li G, Liang Z, Wang X, Yang Y, Shao Z, Li M, Ma Y, Qu F, Morrison DA, Zhang JR (2016). "Addiction of Hypertransformable Pneumococcal Isolates to Natural Transformation for In Vivo Fitness and Virulence". Infect. Immun. 84 (6): 1887–901. doi:10.1128/IAI.00097-16. PMC 4907133 . PMID 27068094.
- ^ Michod RE, Bernstein H, Nedelcu AM (2008). "Adaptive value of sex in microbial pathogens". Infect. Genet. Evol. 8 (3): 267–85. doi:10.1016/j.meegid.2008.01.002. PMID 18295550.
External links[edit] en.wikipedia.org Approach Considerations, Pharmacologic Therapy, Monitoring
Author Zartash Zafar Khan, MD, FACP Infectious Disease Consultant Zartash Zafar Khan, MD, FACP is a member of the following medical societies: American College of Physicians, Infectious Diseases Society of America, International Society for Infectious Diseases Disclosure: Nothing to disclose. Coauthor(s) Michelle R Salvaggio, MD, FACP Assistant Professor, Department of Internal Medicine, Section of Infectious Diseases, University of Oklahoma College of Medicine; Medical Director of Infectious Diseases Institute, Director, Clinical Trials Unit, Director, Ryan White Programs, Department of Medicine, University of Oklahoma Health Sciences Center; Attending Physician, Infectious Diseases Consultation Service, Infectious Diseases Institute, OU Medical Center Michelle R Salvaggio, MD, FACP is a member of the following medical societies: American College of Physicians, Infectious Diseases Society of America Disclosure: Received honoraria from Merck for speaking and teaching. Chief Editor Pranatharthi Haran Chandrasekar, MBBS, MD Professor, Chief of Infectious Disease, Department of Internal Medicine, Wayne State University School of Medicine Pranatharthi Haran Chandrasekar, MBBS, MD is a member of the following medical societies: American College of Physicians, American Society for Microbiology, International Immunocompromised Host Society, Infectious Diseases Society of America Disclosure: Nothing to disclose. Acknowledgements John L Brusch, MD, FACP Assistant Professor of Medicine, Harvard Medical School; Consulting Staff, Department of Medicine and Infectious Disease Service, Cambridge Health Alliance John L Brusch, MD, FACP is a member of the following medical societies: American College of Physicians and Infectious Diseases Society of America Disclosure: Nothing to disclose. Godfrey Harding, MD, FRCP(C) Program Director of Medical Microbiology, Professor, Department of Medicine, Section of Infectious Diseases and Microbiology, St Boniface Hospital, University of Manitoba, Canada Godfrey Harding, MD, FRCP(C) is a member of the following medical societies: American College of Physicians, American Society for Microbiology, Canadian Infectious Disease Society, Canadian Medical Association, Infectious Diseases Society of America, and Royal College of Physicians and Surgeons of Canada Disclosure: Nothing to disclose. Larry I Lutwick, MD Professor of Medicine, State University of New York Downstate Medical School; Director, Infectious Diseases, Veterans Affairs New York Harbor Health Care System, Brooklyn Campus Larry I Lutwick, MD is a member of the following medical societies: American College of Physicians and Infectious Diseases Society of America Disclosure: Nothing to disclose. José Rafael Romero, MD Director of Pediatric Infectious Diseases Fellowship Program, Associate Professor, Department of Pediatrics, Combined Division of Pediatric Infectious Diseases, Creighton University/University of Nebraska Medical Center José Rafael Romero, MD is a member of the following medical societies: American Academy of Pediatrics, American Society for Microbiology, Infectious Diseases Society of America, New York Academy of Sciences, and Pediatric Infectious Diseases Society Disclosure: Nothing to disclose. Mark R Schleiss, MD American Legion Chair of Pediatrics, Professor of Pediatrics, Division Director, Division of Infectious Diseases and Immunology, Department of Pediatrics, University of Minnesota Medical School Mark R Schleiss, MD is a member of the following medical societies: American Pediatric Society, Infectious Diseases Society of America, Pediatric Infectious Diseases Society, and Society for Pediatric Research Disclosure: Nothing to disclose. Sat Sharma, MD, FRCPC Professor and Head, Division of Pulmonary Medicine, Department of Internal Medicine, University of Manitoba; Site Director, Respiratory Medicine, St Boniface General Hospital Sat Sharma, MD, FRCPC is a member of the following medical societies: American Academy of Sleep Medicine, American College of Chest Physicians, American College of Physicians-American Society of Internal Medicine, American Thoracic Society, Canadian Medical Association, Royal College of Physicians and Surgeons of Canada, Royal Society of Medicine, Society of Critical Care Medicine, and World Medical Association Disclosure: Nothing to disclose. Russell W Steele, MD Head, Division of Pediatric Infectious Diseases, Ochsner Children's Health Center; Clinical Professor, Department of Pediatrics, Tulane University School of Medicine Russell W Steele, MD is a member of the following medical societies: American Academy of Pediatrics, American Association of Immunologists, American Pediatric Society, American Society for Microbiology, Infectious Diseases Society of America, Louisiana State Medical Society, Pediatric Infectious Diseases Society, Society for Pediatric Research, and Southern Medical Association Disclosure: Nothing to disclose. Francisco Talavera, PharmD, PhD Adjunct Assistant Professor, University of Nebraska Medical Center College of Pharmacy; Editor-in-Chief, Medscape Drug Reference Disclosure: Medscape Salary Employment Mary L Windle, PharmD Adjunct Associate Professor, University of Nebraska Medical Center College of Pharmacy; Editor-in-Chief, Medscape Drug Reference Disclosure: Nothing to disclose. emedicine.medscape.com In Vitro Antibacterial Activity of Essential Oils against Streptococcus pyogenes
1PRES LUNAM, Université d'Angers, Laboratoire de Bactériologie-Virologie, UFR Sciences Pharmaceutiques et Ingénierie de la Santé, 16 boulevard Daviers, 49045 Angers, Cedex 01, France2PRES LUNAM, Université d'Angers, SFR ICAT 4208, EA 3142 GEIHP, 16 boulevard Daviers, 49045 Angers, Cedex 01, France3S.A. PRANAROM International, 37 Avenue des Artisans, 7822 Ghislenghien, Belgium4PRES LUNAM, Université d'Angers, SFR QUASAV 4207, EA 921 SONAS, UFR Sciences Pharmaceutiques et Ingénierie de la Santé, 16 boulevard Daviers, 49045 Angers, Cedex 01, France Copyright © 2013 Julien Sfeir et al. This is an open access article distributed under the Creative Commons Attribution License, which permits unrestricted use, distribution, and reproduction in any medium, provided the original work is properly cited. Streptococcus pyogenes plays an important role in the pathogenesis of tonsillitis. The present study was conducted to evaluate the in vitro antibacterial activities of 18 essential oils chemotypes from aromatic medicinal plants against S. pyogenes. Antibacterial activity of essential oils was investigated using disc diffusion method. Minimum Inhibitory Concentration of essential oils showing an important antibacterial activity was measured using broth dilution method. Out of 18 essential oils tested, 14 showed antibacterial activity against S. pyogenes. Among them Cinnamomum verum, Cymbopogon citratus, Thymus vulgaris CT thymol, Origanum compactum, and Satureja montana essential oils exhibited significant antibacterial activity. The in vitro results reported here suggest that, for patients suffering from bacterial throat infections, if aromatherapy is used, these essential oils, considered as potential antimicrobial agents, should be preferred. 1. IntroductionSince ten years, the optimization of the use of antibiotics concerns national health agencies that try, through many advertising campaigns and famous slogans, to inform and sensitize people. For example, most cases of tonsillitis are viral and do not need antibiotic treatment. For instance, in about 37% of tonsillitis occurring among children the etiology is bacterial, with Streptococcus pyogenes being the most common bacterial etiology [1]. In this particular context, inflamed tonsils have to be treated by antibiotics. Penicillin is the first choice of antibiotic for the treatment of S. pyogenes tonsillitis. No S. pyogenes resistant to penicillin has been reported. Unfortunately, failures of penicillin treatments to eradicate S. pyogenes from tonsillitis/pharyngitis have been reported [2–4]. Among the alternative therapeutic arsenal, the essential oils (EOs) could be an interesting choice against this pathogen; the EOs antiseptic properties have been demonstrated, at least in vitro (more than 2000 publications about antimicrobial activity of EOs referenced in PubMed since 2002). In spite of all the information available on EOs, we wanted to evaluate their place in alternative or complementary treatments of S. pyogenes tonsillitis. We carried out in vitro experiments to evaluate antibacterial activity of EOs described as active against S. pyogenes. Disc diffusion method was performed to test antibacterial activity of 18 EOs; MIC (Minimum Inhibitory Concentration) and MBC (Minimum Bactericidal Concentration) of the 5 most effective EOs were determined. Similar experiments were carried out with amoxicillin, the benchmark treatment in this pathology. 2. Material and Methods2.1. Essential OilsEighteen essential oils (Cinnamomum verum, Cymbopogon citratus, Origanum compactum, Thymus vulgaris CT thymol, Satureja montana, Eugenia caryophyllus, Cymbopogon martinii var. motia, Cinnamomum camphora CT linalool, Mentha piperita, Thymus vulgaris CT thujanol, Origanum marjorana, Lavandula stoechas, Melaleuca cajuputi, Melaleuca alternifolia, Ocimum basilicum spp. basilicum, Melaleuca quinquenervia CT cineole, Cinnamomum camphora CT cineole, and Rosmarinus officinalis CT cineole) were furnished by Pranarôm Science, France. Major components of these EOs are listed in Table 1. Table 1: Relative percentage composition in the major components for each tested EO according to Pranarôm. 2.2. Bacterial Strains and Culture Conditions Streptococcus pyogenes CIP 104226 strain was used in this study (Collection de l’Institut Pasteur, France). The strain was clinically isolated from pharynx of a child following episode of pharyngitis. 2.3. Disk Diffusion AssayAntimicrobial activity was investigated by disc diffusion method as already described [5]. The bacterial suspension was adjusted to a density of bacterial cells of 1.0 × 108 UFC/mL (or 0.5 McFarland turbidity units). A sterile swab immersed in this bacterial suspension was used to inoculate the entire surface of a sheep blood agar (Biomerieux). 6 L of each EO was applied on a sterile paper disc (Biomerieux) aseptically placed on the inoculated plates. Then, plates were incubated for 15 minutes at room temperature. Only one disc was tested per plate. After 24 h of incubation at 37°C in a CO2 incubator, the inhibition zones were measured in millimetres. Amoxicillin (25 g/disc, Bio-Rad) was used as a positive control for bacterial inhibition. All experiments were done in triplicate. The average of inhibition diameters was calculated to classify the EOs as follows: S. pyogenes is not sensitive (0) for a diameter smaller than 8 mm, moderately sensitive (+) for a 8–14 mm diameter, sensitive (++) for a 14–20 mm diameter, and very sensitive (+++) for a diameter larger than 20 mm [5, 6]. 2.4. Determination of Minimum Inhibitory Concentration and Minimum Bactericidal ConcentrationEssential oils with a large inhibition diameter (>20 mm) were examined for their antimicrobial activity against S. pyogenes. The Minimum Inhibitory Concentration (MIC) was estimated by the broth dilution method in Brain-Heart broth (BH, Biomerieux) using the standardized method described by Courvallin et al. [7]. Briefly, each EO was first diluted in DMSO (diméthylsulfoxyde): 40% (v/v) for Cinnamomum verum and 80% (v/v) for the other EOs tested. Serial dilutions of EOs were carried out in distilled water with concentrations ranging from 0.025% to 1% (v/v), depending on the EO tested. One milliliter of a S. pyogenes inoculum (106 UFC/mL) and 0.1 mL of each EO dilution were added to 2.9 mL of Brain-Heart broth. Controls without EO were prepared. After 24 h of incubation at 37°C under agitation, on hermetic tubes, MIC was determined as the lowest concentration of the EO inhibiting visible bacterial growth. To determine the Minimum Bactericidal Concentration (MBC), 10 L of bacterial inoculum was taken aseptically from tubes that had not presented visible turbidity and was plated onto sheep blood agar [7]. The MBC was considered as the lower concentration of EOs that allowed less than 0.1% of the original inoculum treated with the EO to grow on the surface of the sheep blood agar. Each MIC and MBC value was obtained from three independent experiments. To determine the nature of antibacterial effect of EOs, the MBC/MIC ratio was used; when the ratio was lower than 4, the EO was considered as a bactericidal EO and when the ratio was higher than 4, it was considered as a bacteriostatic EO [8]. 3. Results3.1. Essential Oils CompositionAs depicted in Table 1, essential oils were chosen according to their chemical composition, in particular to their major components. Major compounds of Cinnamomum verum and Cymbopogon citratus were aldehydes. Origanum compactum, Thymus vulgaris CT thymol, Satureja montana, Eugenia caryophyllus, and Ocimum basilicum mainly contained phenolic derivatives. Analysis of Cymbopogon martinii var. motia, Cinnamomum camphora CT linalool, Mentha piperita, Thymus vulgaris CT thujanol, Origanum majorana, and Melaleuca alternifolia indicated terpene alcohols. Ketones were major compounds from Lavandula stoechas. At least, as indicated by their chemotypes, Melaleuca cajuputi, Melaleuca quinquenervia CT cineole, Cinnamomum camphora CT cineole, and Rosmarinus officinalis CT cineole mainly contained cineole, a monoterpene ether. 3.2. Screening of Antibacterial ActivityResults obtained with disk diffusion assay regarding the growth inhibition zones of the tested S. pyogenes strain are presented in Figure 1. Our results showed that EOs from Cinnamomum verum, Cymbopogon citratus, Thymus vulgaris CT thymol, Origanum compactum, and Satureja montana are the most active oils tested against S. pyogenes, with inhibition zones average ranging from 48.0 mm to 35.0 mm (+++). S. pyogenes is sensitive (++) to Eugenia caryophyllus and Cymbopogon martinii var. motia (means inhibition diameters, resp., 18.3 and 15.3 mm). Most of EOs tested showed a moderate inhibitory activity (+) against S. pyogenes (means inhibition diameters ranging from 13.0 to 9.0 mm): Cinnamomum camphora CT linalool, Mentha piperita, Thymus vulgaris CT thujanol, Origanum majorana, Lavandula stoechas, Melaleuca cajuputi, Melaleuca alternifolia. Four EOs showed no significant activity (0) against the tested strain (inhibition zone diameter ranging from 6.3 to 0.0 mm): Ocimum basilicum spp. basilicum, and Melaleuca quinquenervia CT cineole, Cinnamomum camphora CT cineole, and Rosmarinus officinalis CT cineole. Inhibition zones of almost all the essential oils were significantly lower than the positive control amoxicillin (47.3 ± 2.5 mm). Figure 1: Inhibition zone diameters obtained with the various essential oils against S. pyogenes (means ± SD). 3.3. MIC and MBC Values DeterminationReferring to the large inhibition zones observed with disk diffusion method for five essential oils (Cinnamomum verum, Cymbopogon citratus, Thymus vulgaris CT thymol, Origanum compactum, and Satureja montana), the MIC values were determined with broth dilution assays (Figure 2). Cinnamomum verum EO mainly composed of aromatic aldehyde was the most efficient against S. pyogenes (0.19% (v/v)). MIC of Cymbopogon citratus containing mainly terpenic aldehyde was 0.93% (v/v). As far as the EOs rich in aromatic phenols were concerned, MICs ranged from 0.57 to 0.90% (v/v). Figure 2: Minimum Inhibitory Concentrations of five selected essential oils against S. pyogenes (means ± SD). Concerning MBC, in most cases, it was close to the MIC, indicating a good bactericidal activity against S. pyogenes (Table 2), with a ratio of MBC to MIC ranging from 1.02 to 1.53. Table 2: Minimum Bactericidal Concentrations and MBC/MIC ratio of five selected essential oils against S. pyogenes. 4. Discussion Even if aromatic and medicinal plants have been used from ancient times as natural therapies and are considered as alternatives to synthetic drugs, scientific investigations to evaluate antimicrobial activity of essential oils are needed. The aim of this work was to focalize in EOs usable against S. pyogenes and to compare their antimicrobial activity specifically against S. pyogenes, a bacteria responsible for human tonsillitis. Eighteen EOs have been selected for their composition. Indeed, in the literature, it has been reported that EOs containing mainly aromatic phenols or aldehydes presented a major antimicrobial activity against respiratory tract pathogens, followed by EOs containing terpene alcohols. EOs containing terpene ether, ketone, or oxide had weaker activity [9, 10]. Then, for example, thyme, cinnamon, lemongrass, tea tree, lavender, oregano, clove, palmarosa, or cajeput EOs are known to be active against S. pyogenes [9–12] while oregano, basil, mint, rosemary, and lavender EOs are known to inhibit another Gram-positive bacteria, Staphylococcus aureus [13]. In this study, we used the standardized disk assay method to select 5 essential oils showing the higher inhibitory activity against S. pyogenes among 18 essential oils tested. The obtained results, in accordance with the literary works, showed that EOs mainly composed of aldehydes or phenols were the most effective against S. pyogenes. We showed that cinnamon presented the higher activity against S. pyogenes compared to the other EOs tested. These results were consistent with previous works [10, 11]. Therefore, Cinnamomum verum EO containing cinnamaldehyde (an aromatic aldehyde) showed the highest activity. Moreover, EOs containing the aromatic phenols, carvacrol and thymol, were very efficient (+++) against the S. pyogenes strain tested. The other oils containing a phenolic derivative (clove containing eugenol and basil containing estragole) were less active (++). As depicted in Figure 3, these results could be directly linked with the structures of major aromatic phenolic derivatives from EOs. Particularly, the presence of free phenol seems to increase antibacterial activity against S. pyogenes. Basil EO contains mainly estragol without any free phenol. It can be surprising that clove was not selected among the essential oils showing the stronger antibacterial activity. Indeed, previous studies had shown that antibacterial activity of clove EO against S. pyogenes was nearly the same as thyme EO [11]. The differences between our results and previous studies can be due to the fact that the composition of EOs is not strictly defined but is a complex mixture of organic substances, varying in quality and quantity [14]. Figure 3: Structure of phenolic derivatives from tested essential oils. Antibacterial activity of the 5 oils selected was studied by determining the MIC and the MBC. In this study, results of MICs were reliable with diameters of inhibition zones observed with disk diffusion method, with Cinnamomum verum being the more effective EO followed by the other four EOs tested. All tested EOs showed bactericidal activity in vitro (MBCs nearly equal to MICs) but investigations such as pharmacokinetic and pharmacodynamic studies are needed to characterize the antibacterial activity in vivo and their clinical efficacy [15]. The comparison of antibacterial activity and cytotoxicity of EOs and antibiotics must be approached with caution. Indeed, due to the complex and variable chemical composition of EOs, affected by many factors like chemotypes or cultivation conditions, it is still difficult to understand antibacterial activity mechanisms of EOs and to control their cytotoxicity. As discussed later, at least one part of the antibacterial and cytotoxic activities of essential oils is nonspecific but linked with lipophilic compounds targeting membranes. Screening the EOs presenting antibacterial effects, Fabio et al. have reported that MICs of the EOs showing antibacterial activity were higher than the nontoxic concentrations on Vero cells [11]. Investigations on cytotoxicity of EOs have to be conducted, particularly in terms of possibility of overdoses and in terms of interactions with drugs. Moreover, the lack of clinical studies (toxicity, pharmacokinetic, etc.) has to be underlined. Therapeutic doses of EOs containing phenols or aldehydes are usually only a few drops per day (2 drops 3 times a day) per os. The inhalation of such irritating EOs should be avoided. It should be noticed that pharmacokinetic predictions about mixtures such as EOs are difficult [14]. Nevertheless, as essential oils are lipophilic and volatile compounds, they can rapidly reach the systemic compartment and be partially eliminated through respiratory way, that is, on the infectious site in tonsillitis context [16]. Antibacterial treatment of S. pyogenes tonsillitis has several objectives; among them is the reduction of the transmission to family members. Indeed, it seems that there is an increased risk concerning the invasive infections (e.g., bacteraemia and pneumonia) for household contacts of index patients, compared to the annual incidence rate of sporadic invasive infections caused by this bacteria [17]. However, about 20–30% of antibiotic treatments with penicillin fail to eradicate the pathogen [3, 4]. This failure in therapy is not due to a resistant phenotype to penicillin [18] but can be related to various hypothesis such as microbiologic interactions between commensal pharyngotonsillar flora and S. pyogenes, poor penetration and diffusion of penicillin into tonsils, or reacquisition of S. pyogenes from a contact [2]. In this context, synergy of action of EOs with antibiotics could be investigated. With a nonspecific mode of action, EOs could help to control beta-lactamase-producing bacteria causing failure of penicillin treatment to eradicate S. pyogenes (for a review see [19]). An illustration of this promising strategy of combination is the study performed by Fadli et al. Among 80 EOs/antibiotics combinations tested, 71% showed synergism. For example, it has been noted that carvacrol showed a synergistic effect when combined with ciprofloxacin [20]. Moreover, this alternative strategy could be interesting because it can ideally lead to a reduction of doses of the antibiotics, thus reducing the adverse effects of the therapy. However, the synergistic effects have to be evaluated in vitro and in vivo as effects are variable, depending on many factors like EO composition, exposure time, or mode of action of the active components of the EO. Investigations on EOs mechanisms of action have not been fully established yet. Studies on Cinnamomum sp. and on its major components showed morphological changes (loss of membrane integrity) on Gram-positive bacteria (Staphylococcus aureus or Bacillus cereus) and a decrease in the metabolic activity and in the bacterial replication capacity [21, 22]. Carvacrol exposure causes morphological modifications, as changes in cell surface structure [23, 24]. However, as EOs components are lipophilic, membranes of various organisms are likely targeted but protein targets seem nonspecific; particularly, phenolic alcohols or aldehydes interfere with membrane-integrated or -associated enzyme proteins, stopping their activity. Components of EOs can also interfere with electron transport chain from bacterial or mammalian mitochondria and alter energy production [25]. 5. ConclusionIn conclusion, we show an interesting antibacterial activity of some essential oils against S. pyogenes, particularly Cinnamomum verum EO but we need further investigations to evaluate bactericidal properties in practical applications on clinical strains and to assess the potential for therapeutic application. Fronting the fact that there is no evidence of a potential clinical use of these EOs, further researches are needed in order to determine if they could substitute efficiently antibiotics or, perhaps, be used in combination. Additional in vivo studies and clinical trials would help to justify and evaluate the potential of these oils in tonsillitis context. www.hindawi.com Virulence factors of Streptococcus pyogenes and their roles
Impetigo Streptococcus pyogenes is the leading cause of uncomplicated bacterial pharyngitis and tonsillitis commonly referred to a strep throat. Acute diseases associated with Streptococcus pyogenes occur chiefly in the respiratory tract (sinusitis, otitis), bloodstream (sepsis, endocarditis, meningitis), or the skin {impetigo, cellulitis, necrotizing fasciitis , myositis}. Two nonsuppurative post streptococcal sequelae, rheumatic fever and glomerulonephritis, may follow 1-3 weeks after acute illness. Note: S. pyogenes is also designated as “flesh-eating bacteria” because of its characteristics skin infections with involvements of deeper tissues and organs. Mnemonic: Virulence factors of Streptococcus pyogenes: “SMASHED”StreptolysinsM proteinAnti-C5a peptidaseStreptokinaseHyaluronidase and Hyaluronic acid CapsuleExotoxinDNAses Cell surface structure and virulence factors of S. pyogenesWide variety of virulence factors help Streptococcus pyogenes to achieve this success, by increasing it’s ability to colonize, multiply, evade host immune response and spread in it’s host. Exotoxins, structural components and enzymes that contribute to its virulence are: - Hyaluronic Acid CapsuleCapsules are major virulence factors of many pathogenic bacteria as they are anti-phagocytic in nature. The hyaluronic acid capsule of S. pyogenes is non antigenic because of its chemical similarity to host connective tissue. Capsule of S. pyogenes thus assists the bacterium to hide its own antigens and to go unrecognized as antigenic by its host. The hyaluronic acid capsule also prevents opsonized phagocytosis.
- Adhesins: Adhesion of S. pyogenes in the epithelial cells of oral, nasal cavities and skin is the first step for infection. S. pyogenes produces various adhesins; Fibronectin (Fn) binding protein, lipoteichoic acids (LTA), M protein, Protein F etc.
- M ProteinM protein is the major virulence factor of group A beta hemolytic streptococci. It has anti-phagocytic and anti-complement properties. It is cytotoxic for neutrophils. Streptococcal M protein mimic those of mammalian muscle and connective tissue. More than 50 types of S. pyogenes M proteins have been identified on the basis of antigenic specificity.
- The M proteins of lower M-types (e.g., 1, 3, 5, 6, 14, 18, 19, 24) are considered rheumatogenic since they contain antigenic epitopes related to heart muscle, and may lead to autoimmune rheumatic carditis (rheumatic fever) following an acute pharyngeal infection.
- Acute glomerulonephritis can follow streptococcal infection of the pharynx (pharyngitis-associated Acute glomerulonephritis e.g., 1, 4, 12, 25) or or the skin (pyoderma-associated AGN-mainly higher M types e.g., 2, 49, 55, 57, 59, 60, 61) is caused by nephritogenic strains.
- Hemolysins: Two hemolytic and cytolytic toxins-streptolysin O (SLO) and Streptolysin S (SLS) are produced by most strains of group A streptococci.
Blood agar showing β-hemolysis- Streptolysin O: The oxygen labile cytolysin is responsible for beta hemolysis seen around Group A streptococcus (GAS) colonies in blood agar. It is believed to be toxic to leukocytes. Streptolysin O is strongly immunogenic. The concentration of antistreptolysin O antibodies is used diagnostically as an indicator of prior or recent streptococcal infection.
- Streptolysin S: It is oxygen stable and non-immunogenic cytolysin. However, like streptolysin O, streptolysin S is hemolytic and cytotoxic.
- Pyrogenic Exotoxins of Streptococcus pyogenes (SPEs) types A, B and CThese toxins acts as superantigens (not requiring processing by antigen presenting cells). SPEs A and C are produced when S. pyogenes is lysogenized strains (bacteria carrying an integrated phage i.e. prophage). Non lysogenized strains are atoxic. SPE B is encoded by a bacterial chromosome.These toxins act directly on the hypothalamus to exert their pyrogenic (fever producing) properties, and they cause the rash characteristics of scarlet fever. Another disease mediated by production of potent SPE is Streptococcal toxic shock syndrome, typified by multi-system involvement that includes renal and respiratory failure, rash and diarrhea.
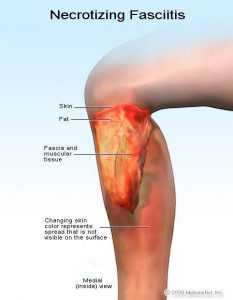 - Spreading factors: These are enzymes that help streptococci to invade tissues by dissolving tissue clots and destroying connective tissue. Enzymes in this group includes:
- Deoxyribonucleases (streptodornase DNase): These enzymes depolymerize free DNA present in the pus. There are four antigenically distinct nucleases (A, B, C and D), of which B is the most antigenic to humans. Streptodornases A-D possess deoxyribonuclease activity; Streptodornases B and D possess ribonuclease activity as well. Antibody titers to DNase B are of great importance in the sero-diagnosis of pharyngeal or skin infections.
- Streptokinase (fibrinolysin)Streptokinase acts on plasminogen, which is converted to plasmin, an active proteolytic enzymes that lyse fibrin. Thus streptokinase appears to play a biological role in streptococcal infections by breaking down the fibrin barrier around the lesions and facilitates the spread of infection. Antistreptokinase antibodies provide retrospective evidence of streptococcal infection.
- Hyaluronidase: Hyaluronidase splits hyaluronic acid, an important component of connective tissue. Thus hyaluronidase aids in spreading of the microorganisms along the intercellular spaces.
- Other enzymes: Many strains of streptococci also produce Proteases (proteolytic degradation of host immune effector molecules eg, antibodies, complement etc), Serum Opacity Factor (SOF), Nicotinamide Adenine Dinucleotidase (NADase), ATPase, phosphatase, esterases, amylase, N-acetylglucosaminidase, neuraminidase and other enzymes which plays major role in pathogenesis.
Related Tankeshwar Acharya Hello, thank you for visiting my blog. I am Tankeshwar Acharya. Blogging is my passion, I am working as a Asst. Professor and Microbiologist at Department of Microbiology and Immunology, Patan Academy of Health Sciences, Nepal. If you want me to write about any posts that you found confusing/difficult, please mention in the comments below. microbeonline.com Streptococcus pyogenes and streptococcal disease
Streptococcus pyogenes and Streptococcal Disease (page 2)
(This chapter has 4 pages)
© Kenneth Todar, PhD
Pathogenesis
Streptococcus pyogenes owes its major success as a pathogen
to
its ability to colonize and rapidly multiply and spread in its host
while
evading phagocytosis and confusing the immune system.
Acute diseases associated with Streptococcus pyogenes
occur chiefly in the respiratory tract, bloodstream, or
the
skin. Streptococcal disease is most often a respiratory infection
(pharyngitis
or tonsillitis) or a skin infection (pyoderma). Some strains of
streptococci
show a predilection for the respiratory tract; others, for the skin.
Generally,
streptococcal isolates from the pharynx and respiratory tract do not
cause
skin infections. Figure 3 describes the pathogenesis of S. pyogenes
infections.
S. pyogenes is the leading cause of uncomplicated bacterial pharyngitis
and tonsillitis commonly referred to a strep throat.
Other
respiratory infections include sinusitis,
otitis, and pneumonia.
Infections of the skin can be superficial (impetigo) or deep
(cellulitis). Invasive streptococci cause joint or bone
infections,
destructive wound infections (necrotizing fasciitis) and
myositis,
meningitis
and
endocarditis. Two post streptococcal sequelae,
rheumatic
fever and glomerulonephritis, may follow streptococcal
disease,
and occur in 1-3% of untreated infections. These conditions and their
pathology
are not attributable to dissemination of bacteria, but to aberrent
immunological
reactions to Group A streptococcal antigens.
Scarlet fever and streptococcal
toxic shock syndrome are systemic responses to circulating
bacterial
toxins.
The cell surface of Streptococcus pyogenes accounts
for
many of the bacterium's determinants of virulence, especially those
concerned
with colonization and evasion of phagocytosis and the host immune
responses.
The surface of Streptococcus pyogenes is incredibly complex and
chemically-diverse. Antigenic components include capsular
polysaccharide
(C-substance), cell wall peptidoglycan and lipoteichoic
acid
(LTA), and a variety of surface proteins, including M protein,
fimbrial
proteins, fibronectin-binding proteins, (e.g. Protein F)
and cell-bound streptokinase.
The cytoplasmic membrane of S. pyogenes contains some
antigens
similar to those of human cardiac, skeletal, and smooth muscle, heart
valve
fibroblasts, and neuronal tissues, resulting in molecular mimicry
and a tolerant or suppressed immune response by the host.
The cell envelope of a Group A streptococcus is illustrated in
Figure
2. The complexity of the surface can be seen in several of the electron
micrographs of the bacterium that accompany this article.
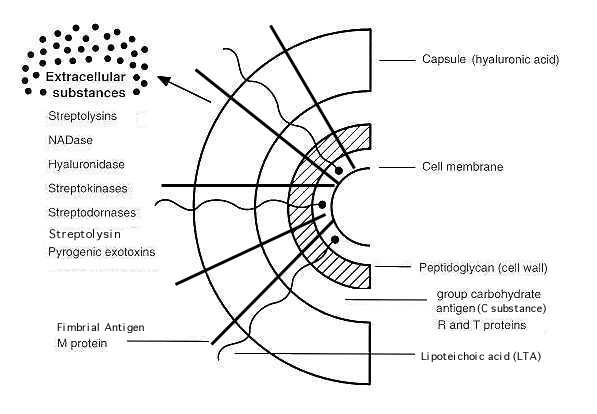
Figure 2. Cell surface
structure
of Streptococcus pyogenes and secreted products involved in
virulence.
In Group A streptococci, the R and T proteins are
used
as epidemiologic markers and have no known role in virulence. The group
carbohydrate antigen (composed of N-acetylglucosamine and
rhamnose)
has been thought to have no role in virulence, but emerging strains
with
increased invasive capacity produce a very mucoid colony, suggesting a
role of the capsule in virulence.
The M proteins are clearly virulence factors associated with
both colonization and resistance to phagocytosis. More than 50
types
of S. pyogenes M proteins have been identified on the basis of
antigenic
specificity, and it is the M protein that is the major cause of
antigenic
shift and antigenic drift in the Group A streptococci. The M protein
(found
in fimbriae) also binds fibrinogen from serum and blocks the binding of
complement to the underlying peptidoglycan. This allows survival of the
organism by inhibiting phagocytosis.
The streptococcal M protein, as well as peptidoglycan,
N-acetylglucosamine,
and group-specific carbohydrate, contain antigenic epitopes that mimic
those of mammalian muscle and connective tissue. As mentioned above,
the
cell surface of recently emerging strains of streptococci is distinctly
mucoid (indicating that they are highly encapsulated). These strains
are
also rich in surface M protein. The M proteins of certain M-types are
considered
rheumatogenic
since they contain antigenic epitopes related to heart muscle, and they
therefore may lead to autoimmune rheumatic carditis (rheumatic fever)
following
an acute infection.
The Hyaluronic Acid Capsule
The capsule of S. pyogenes is non antigenic since it
is
composed of hyaluronic acid, which is chemically similar to
that
of host connective tissue. This allows the bacterium to hide its own
antigens
and to go unrecognized as antigenic by its host. The Hyaluronic acid
capsule
also prevents opsonized phagocytosis by neutrophils or mancrophages.
Adhesins
Colonization of tissues by S. pyogenes is thought to result
from
a failure in the constitutive defenses (normal flora and other
nonspecific
defense mechanisms) which allows establishment of the bacterium at a
portal
of entry (often the upper respiratory tract or the skin) where the
organism
multiplies and causes an inflammatory purulent lesion.
It is now realized that S. pyogenes (like many other
bacterial
pathogens) produces multiple adhesins with varied specificities. There
is evidence that Streptococcus pyogenes utilizes lipoteichoic
acids (LTA), M protein, and multiple fibronectin-binding
proteins in its repertoire of adhesins. LTA is anchored to proteins
on the bacterial surface, including the M protein. Both the M proteins
and lipoteichoic acid are supported externally to the cell wall on
fimbriae
and appear to mediate bacterial adherence to host epithelial
cells.
The fibronectin-binding protein, Protein F, has also been shown
to mediate streptococcal adherence to the amino terminus of fibronectin
on mucosal surfaces.
Identification of Streptococcuspyogenes adhesins has long
been
a subject of conflict and debate. Most of the debate was between
proponents
of the LTA model and those of the M protein model. In 1972, Gibbons and
his colleagues proposed that attachment of streptococci to the oral
mucosa
of mice is dependent on M protein. However, Olfek and Beachey argued
that
lipoteichoic acid (LTA), rather than M protein, was responsible for
streptococcal
adherence to buccal epithelial cells. In 1996, Hasty and Courtney
proposed
a two-step model of attachment that involved both M protein and
teichoic
acids. They suggested that LTA loosely tethers streptococci to
epithelial
cells, and then M protein and/or other fibronectin (Fn)-binding
proteins
secure a firmer, irreversible association. The first streptococcal
fibronectin-binding
protein (Sfb) was demonstrated in 1992. Shortly thereafter, protein F
was
discovered. Most recently (1998), the M1 and M3 proteins were
shown
to bind fibronectin.
Extracellular products: invasins and
exotoxins
Colonization of the upper respiratory tract and acute pharyngitis
may
spread to other portions of the upper or lower respiratory tracts
resulting
in infections of the middle ear (otitis media), sinuses (sinusitis), or
lungs (pneumonia). In addition, meningitis can occur by direct
extension
of infection from the middle ear or sinuses to the meninges or by way
of
bloodstream invasion from the pulmonary focus. Bacteremia can also
result
in infection of bones (osteomyelitis) or joints (arthritis). During
these
aspects of acute disease the streptococci bring into play a variety of
secretory proteins that mediate their invasion.
For the most part, streptococcal invasins and protein toxins
interact
with mammalian blood and tissue components in ways that kill host cells
and provoke a damaging inflammatory response. The soluble extracellular
growth products and toxins of Streptococcus pyogenes (see
Figure
2, above), have been studied intensely. Streptolysin S is an
oxygen-stable
leukocidin; Streptolysin O is an oxygen-labile leukocidin.
NADase
is also leukotoxic. Hyaluronidase (the original
"spreading
factor") can digest host connective tissue hyaluronic acid, as well as
the organism's own capsule. Streptokinases participate in
fibrin
lysis. Streptodornases A-D possess deoxyribonuclease activity;
Streptodornases
B and D possess ribonuclease activity as well. Protease
activity
similar to that in Staphylococcus aureus has been shown in
strains
causing soft tissue necrosis or toxic shock syndrome. This large
repertoire
of products is important in the pathogenesis of S. pyogenes
infections.
Even so, antibodies to these products are relatively insignificant in
protection
of the host.
The streptococcal invasins act in a variety of ways summarized in
Table
1 at the end of this article. Streptococcal invasins lyse eukaryotic
cells,
including red blood cells and phagocytes; they lyse other host
macromolecules,
including enzymes and informational molecules; they allow the bacteria
to spread among tissues by dissolving host fibrin and intercellular
ground
substances.
Pyrogenic Exotoxins
Three streptococcal pyrogenic exotoxins (SPE), formerly
known
as Erythrogenic toxin, are recognized: types A, B, C. These
toxins
act as superantigens by a mechanism similar to those described
for
staphylococci. As antigens, they do not requiring processing by antigen
presenting cells. Rather, they stimulate T cells by binding class II
MHC
molecules directly and nonspecifically. With superantigens about 20% of
T cells may be stimulated (vs 1/10,000 T cells stimulated by
conventional
antigens) resulting in massive detrimental cytokine release. SPE A and
SPE C are encoded by lysogenic phages; the gene for SPE B is located on
the bacterial chromosome.
The erythrogenic toxin is so-named for its association with scarlet
fever which occurs when the toxin is disseminated in the blood.
Re-emergence
in the late 1980's of exotoxin-producing strains of S. pyogenes
has been associated with a toxic shock-like syndrome similar in
pathogenesis and manifestation to staphylococcal toxic shock syndrome,
and with other forms of invasive disease associated with severe tissue
destruction. The latter condition is termed necrotizing fasciitis.
Outbreaks of sepsis, toxic shock and necrotizing fasciitis have been
reported
at increasing frequency. The destructive nature of wound infections
prompted
the popular press to refer to S. pyogenes as "flesh-eating
bacteria"
and "skin-eating streptococci". The increase in invasive
streptococcal
disease was associated with emergence of a highly virulent serotype
M1
which is disseminated world-wide. The M1 strain produces the
erythrogenic
toxin (Spe A), thought to be responsible for toxic shock, and the
enzyme cysteine protease which is involved in tissue destruction.
Because clusters of toxic shock were also associated with other
serotypes,
particularly M3 strains, it is believed that unidentified host factors
may also have played an important role in the resurgence of these
dangerous
infections.
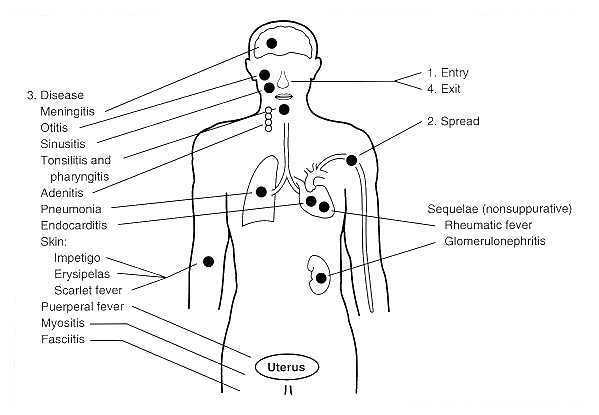
FIGURE 3. Pathogenesis of Streptococcus
pyogenes infections. Adapted from Baron's Medical
Microbiology
Chapter 13, Streptococcus
by Maria Jevitz Patterson.
www.textbookofbacteriology.net What are Streptococcal infections? | Facts
Streptococcal infections are any type of infection caused by the group of bacteria Streptococcus. - There are many different types of Streptococci and infections vary in severity from mild throat infections to pneumonia.
- Streptococcal infections are primarily treated with antibiotics.
- Streptococci are divided into two key groups:
- alpha (α)-haemolytic Streptococci
- beta (β)-haemolytic Streptococci.
α-haemolytic Streptococci - This group is very common.
- Many strains live naturally in humans causing no symptoms.
- α-haemolytic Streptococci are split into two groups:
- Streptococcus pneumoniae
- Viridans Streptococci.
Streptococcus pneumoniae - S. pneumoniae is usually found on the surface of the skin and inside the throat.
- It causes infections in both adults and children.
- It is transmitted through coughs and sneezes.
- Minor infections can be treated relatively easily with antibiotics and include:
- sinusitis (inflammation of the sinuses)
- middle ear infections.
- More invasive infections pose a more serious threat to health and include:
- pneumonia (inflammation of the tissue in the lungs)
- meningitis (inflammation of the membranes covering the brain and spinal cord)
- bacteraemia (infection of the blood).
- People at the highest risk of invasive S. pneumoniae infections are:
- babies under six months old
- adults over 75 years old
- adults with a weak immune system.
Viridans Streptococci - This group of Streptococci are most often found in the mouth, gut and genital region.
- The most serious Viridans infections occur when the bacteria enters other regions of the body. For example, if Viridans gets into the bloodstream it can cause endocarditis (infection of the inner lining of the heart).
- Individuals with damaged heart valves or cardiac abnormalities and compromised immune systems are at particular risk.
- Symptoms include:
- tiredness
- weakness
- fever
- weight loss
- respiratory problems
- problems with heart function in cases where endocarditis occurs.
Diagnosis and treatment of α-haemolytic infections - Minor infections can be diagnosed by taking a sample of saliva or a swab of the affected tissue and testing for the presence of Streptococcal bacteria.
- For invasive infections additional tests may be required, such as a blood test for bacteraemia or cerebral spinal fluid test for meningitis.
- Minor infections may not require treatment or may be treated with antibiotics.
- Invasive infections usually result in a hospital stay.
- Severe invasive infections may require intensive treatment with intravenous antibiotics for 7-10 days. In some cases surgery may be required to remove or repair damaged tissue.
β-haemolytic Streptococci - β-haemolytic Streptococci are characterised as Group A Streptococci (GAS) and Group B Streptococci (GBS)
Group A (Streptococcus pyogenes) - Streptococcus pyogenes is transmitted through coughs, sneezes or direct contact
- It can be either non-invasive (not spread into the bloodstream) or invasive (spread into the bloodstream and to other body sites)
- The most common of the non-invasive infections include:
- strep throat: sore throat specifically caused by streptococcal infection
- impetigo: contagious skin infection that causes sores and blisters
- scarlet fever: infectious disease causing a sore throat and characteristic red rash.
- Invasive infections are much rarer and occur when the bacteria infects other areas of the body such as the blood and organs. This can result in:
- pneumonia: inflammation of the tissue in the lungs
- bacteraemia: infection of the blood
- necrotising fasciitis: flesh-eating disease.
- Left untreated, potential complications of Group A infection include rheumatic fever, a disease that affects the joints, kidneys and heart.
Diagnosis and treatment of Group A infections - For non-invasive infections a Rapid Strep Test (RST) can be carried out. This involves a doctor taking a throat or nose swab that is analysed for group A Streptococcus. An RST is one of the most common tests for this type of infection.
- For suspected invasive infections a blood test may be taken.
- Most infections are treated with antibiotics:
- superficial skin infections can be treated with topical antibiotic ointments
- other infections can be treated with oral or intravenous antibiotics depending on the severity of the infection.
- Where the infection has caused a lot of skin damage, for example in cases of necrotising fasciitis, damaged tissue may be removed surgically.
Group B (Streptococcus agalactiae) - Streptococcus agalactiae usually lives harmlessly inside the digestive system and female genitals.
- It can be transmitted sexually or from mother to baby during birth.
- Group B β-haemolytic Streptococcus tend to only affect new born babies as the bacteria can be passed onto the baby from the mother through the amniotic fluid (protective fluid that surrounds the foetus in the womb).
- Due to being continually exposed to it through our lifetimes most people quickly develop a natural immunity to Group B β-haemolytic Streptococcus.
- Symptoms of group B β-haemolytic streptococcal infection in a newborn baby include:
- being floppy and unresponsive
- poor feeding
- unusually high or low body temperature
- unusually fast or slow heart rate
- If left untreated, group B β-haemolytic streptococcal infections can also lead to much more serious conditions such as meningitis and pneumonia.
- Factors increasing risk of group B β-haemolytic streptococcal infection in new-born babies include:
- premature birth
- being part of a multiple birth
- having a mother with a history of group B streptococcal infection.
Diagnosis and treatment of Group B infections - These infections are diagnosed by a blood, urine or cerebral spinal fluid sample to identify the bacteria.
- A culture of fluid from the vagina or rectum can also determine if a woman is infected.
- The infection is treated with antibiotics, often directly into the bloodstream (intravenously).
- Treatment of these infections may require a hospital stay.
Preventing Group B Streptococcal infection in newborns - Healthcare professionals take measures during labour and after birth to prevent newborns becoming infected with Streptococcus.
- If a baby is known to be at risk of Streptococcus infection, antibiotic injections are given to the mother during labour or to the baby shortly after birth.
This page was last updated on 2015-06-19 www.yourgenome.org
|